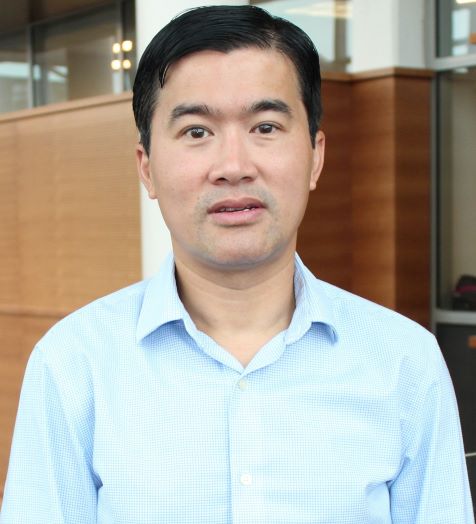
Hien Nguyen
Carl Johnson/Pfizer Professor of Organic Chemistry
313-577-2557
313-577-8822 (fax)
Chem 337/341
Website(s)
Department
ChemistryHien Nguyen
Research interest(s)/area of expertise
Cancer Drug Discovery, Vaccine Adjuvant Immunotherapy, Alzheimer's Disease, Chemical Biology, Glycobiology, Catalytic Carbohydrate Methods, Transition-Metal Catalysis, Fluorination and Radiofluorination for PET Imaging.
Research
Hien M. Nguyen was born in Ho Chi Minh City (Saigon), Vietnam. Prof. Nguyen came to the US in 1989 and first settled with his family in Boston, Massachusetts. He graduated with honors from Tufts University in Medford, Massachusetts where he majored in chemistry and conducted research with Professor Marc d'Alarcao. Hien left Boston and moved to attend the University of Illinois at Urbana-Champaign and he subsequently joined the group of the late David Y. Gin.
His Ph.D. thesis focused on developing new carbohydrate methodologies for efficient assembly of complex oligosaccharides. As an NIH postdoctoral fellow in the group of Prof. Barry Trost at Stanford University, Prof. Nguyen pursued training in the area of transition-metal catalysis. Prof. Nguyen began his independent career in 2006 and was promoted with tenure at the University of Iowa. In 2018, he relocated to Wayne State University as the Carl Johnson/Pfizer endowed chair and professor of chemistry.
One of the major research programs in the laboratory of Prof. Nguyen is to develop heparan sulfate mimetics for studying cancer metastasis, tumor cell invasion, diabetes, Alzheimer's disease and SARS-CoV-2 infection. The second program invovles structure-based design of carbohydrate vaccine adjuvants for immunotheraphy. The third research program involves the development of a series of catalytic stereoselective glycosylation methodologies that can be used by non-specialists for the efficient assembly of bioactive carbohydrate and glycopeptide molecules. In particular, we exploit phenanthroline and protonated phenanthroline as the catalysts to selectively promote the formation of high purity glycosides in high yields and with excellent selectivity.
We also invovle the development of novel methodologies for the enantioselective construction of C-F, C-O and C-N containing compounds that possess significant bioactivity utilizing transition-metal-catalyzed processes. The methods will be applied to the synthesis of bioactive molecules, setting the stage for subsequent biological studies as well as the design and development of related structural analogs. Recently, we have developed a rapid and mild conditions for fluorine-18 incorporation into small organic molecules for potential use as PET radiotracers.
Education
- B.S., Tufts University, 1996
- Ph.D., University of Illinois at Urbana-Champaign, 2003
- NIH Postdoctoral Fellow, Stanford University, 2003 - 2006
Awards and grants
(1) R01GM098285 (06/01/2019 - 05/31/2024) "Tailoring Structure of Sulfated Oligosaccharides for Modulating Heparanase Activity"
(2) R01GM136968 (04/01/2020 - 03/31/2024) "Strategies for Expedited Synthesis of Sulfated Aminoglycans"
(3) R01AI169505 (06/01/2022 - 05/31/2027) "Synthesis and Evaluation of Carbohydrate Vaccine Adjuvants"
(4) R35GM149213 (09/01/2023 - 08/31/2018) "Development of Catalytic Glycosylations and Biologically Important Glycosaminoglycans"
Selected publications
- Wakpal, J.; Pathiranage, P.; Walker, A.; Nguyen, H. M. "Rational Design and Expedient Synthesis of Heparan Sulfate Mimetics from Natural Aminoglycosides for Structure and Activity Relationship Studies" Angew. Chem. Int. Ed. 2023, 62, e2020304325.
- Usman, F.; Gogoi, A. R.; Mixdorf, J. C.; Gutierrez, O.; Nguyen, H. M. "Rhodium-Catalyzed Asymmetric Synthesis of 1,2-Disubstituted Allylic Fluorides." Angew. Chem. Int. Ed. 2023, 62, e202314843.
- Rodrigo, S.; Hazra, A.; Mahajan, J. P.; Nguyen, H. M.; Luo, L. "Overcoming the Potential Window-Limited Functional Group Compatibility by Alternating Current Electrolysis." J. Am. Chem. Soc. 2023, 145, 21851-21859.
- Arachchi, M. K.; Schaugaard, R. N.; Schlegel, H. B.; Nguyen, H. M. "Asymmetric Synthesis of alpha-Trisubstituted-alpha-Tertiary Amines via Rhodium-Catalysis." J. Am. Chem. Soc. 2023, 145, 19642-19654.
- Abdulsalam, H.; Li, J.; Loka, R. S.; Sletten, E. T.; Nguyen, H. M. "Heparan Sulfate Mimicking Glycopolymers Bind SARS-CoV-2 Spike Protein in a Length and Sulfation Pattern Dependent Manner" ACS Med. Chem. Lett. 2023, 14, 1411-1418.
- Li, J.; Nguyen, H. M. "Phenanthroline Catalysis in Stereoselective 1,2-cis Glycosylations." Acc. Chem. Res. 2022, 55, 3738-3751.
- Loka, R. S.; Song, Z.; Sletten, E. T.; Kayal, Y.; Vlodavsky, I.; Zhang, K.; Nguyen, H. M. "Heparan Sulfate-Like Glycopolymer Prevents Pancreatic Beta Cell Destruction and Suppresses Islet Inflammation under the Challenge of Upregulated Heparanase." ACS Chem. Biol. 2022, 17, 1387-1400.
- Xu, H.; Schaugaard, R. N.; Li, J.; Schlegel, H. B.; Nguyen, H. M. "Phenanthroline-Catalyzed Stereoselective 1,2-cis Furanosylations." J. Am. Chem. Soc. 2022, 144, 7441-7456.
- Arachchi, M. K.; Nguyen, H. M. "Iridium-Catalyzed Enantioselective Allylic Substitutions of Racemic Branched Trichloroacetimidates with Hetereoatom Nucleophiles." Adv. Synth. Catal. 2021, 363, 4239-4246.
- Schaugaard, R. N.; Nguyen, H. M.; Schlegel, H. B. "Alkyl Radical-Free Cu(II) Photocatalytic Cross-Coupling: A Theoretical Study of Anomerically Specific Photocatalyzed Glycosylation of Pyranosyl Bromide." Inorg. Chem. 2021, 60, 12801-12812.
- Li, J.; Nguyen, H. M. "A Mechanistic Probe into 1,2-cis Glycoside Formation Catalyzed by Phenanthroline and Further Expansion of Scope." Adv. Synth. Catal. 2021, 363, 4054-4066.
- DeMent, P. M.; Lu, C.; Wakpal, J.; Schaugaard, R. N.; Schlegel, H. B.; Nguyen, H. M. "Phenanthroline-Catalyzed Stereoselective Formation of alpha-1,2-cis-2-Deoxy-2-Fluoro Glycosides." ACS Catalysis, 2021, 11, 2108-2120.
- Vlodavsky, I.; Barash, U.; Nguyen, H. M.; Yang, S.-M.; Ilan, N. "Biology of Heparanase-Heparan Sulfate Axis and Its Role in Disease Pathogenesis." Sem. Thromosis Hemostasis 2021, 47, 240-253.
- Zhu, S.; Li, J.; Loka, R. S.; Vlodavsky, I.; Zhang, K.; Nguyen, H. M. “Modulating Heparanase Activity: Tuning Sulfation Pattern and Glycosidic Linkage of Oligosaccharides” J. Med. Chem. 2020, 63, 4227-4255.
- Zhang, K.; Liu, H.; Song, Z.; Jiang, Y.; Kim, H.; Samavati, L.; Nguyen, H. M.; Yang, Z.-Q. "The UPR Trasducer IRE1 Promotes Breast Cancer Malignancy by Degrading Tumor Suppressor microRNAs." iScience 2020, 23, 101503.
- Rodrigo, S.; Um, C.; Mixdorf, J. C.; Gunasekera, D.; Nguyen, H. M.; Long, L. "Alternating Current Electrolysis for organic Electrosynthesis: Trifluoromethylation of (Hetero)arenes." Org. Lett. 2020, 22, 6719-6723.
- Yu, F.; Dickson, J. L.; Loka, R. S.; Xu, H.; Schaugaard, R. N.; Schlegel, H. B.; Luo, L.; Nguyen, H. M. "Diastereoselective sp3 C-O Bond Formation via Visible Light-Induced Copper-Catalyzed Cross Couplings of Anomeric Alkyl Bromides with Aliphatic Alcohols." ACS Catalysis, 2020, 10, 5990-6001.
- Sorlin, A. M.; Fuad, U. O.; English, C. K.; Nguyen, H. M. "Advances in Nucleophilic Allylic Fluorination." ACS Catalysis 2020, 10, 11980-12010.
- Loka, R. S.; Sletten, E. T.; Barash, U.; Vlodavsky, I.; Nguyen, H. M. “Specific Inhibition of Heparanase by Glycopolymer with Well-Defined Sulfation Pattern Prevents Breast Cancer Metastasis in Mice.” ACS Appl. Mater. Interfaces 2019, 11, 244-254.
- Yu, F.; Li, J.; DeMent, P. M.; Tu, Y.-J.; Schlegel, H. B.; Nguyen, H. M. “Phenanthroline-Catalyzed Stereoselective Glycosylations.” Angew. Chem. Int. Ed. 2019, 58, 6957-6961.
- Sletten, E. T.; Tu, Y.-T.; Schlegel, H. B.;* Nguyen, H. M.* “Are Brønsted Acids the True Promoter of Metal Triflate Catalyzed Glycosylations? A Mechanistic Probe into 1,2-cis-Aminoglycoside Formation by Nickel Triflate.” ACS. Catalysis 2019, 9, 2110-2123.
- Zhu, S.; Samala, G.; Sletten, E. T.; Stockdill, J. L.; Nguyen, H. M. “Facile Triflic Acid-Catalyzed a-1,2-cis-Thiol Glycosylations: Scope and Application to the Synthesis of S-Linked Oligosaccharides, Glycolipids, Sublancin Glycopeptides, and TN/TF Antigens.” Chem. Sci. 2019, 10, 10475-10480.
- Sorlin, A. M.; Mixdorf, J. C.; Rotella, M.; Martin, R.; Gutierrez, O.; Nguyen, H. M. “The Role of Trichloroacetimidate to Enable Irididium-Catalyzed Regio- and Enantioselective Allylic Fluorination.” J. Am. Chem. Soc. 2019, 143, 14843-14882.
- Mixdorf, J. C; Sorlin, A. M.; Dick, D.; Nguyen, H. M. “Iridium-Catalyzed Radiosynthesis of Branched Allylic [18F]Fluorides.” Org. Lett. 2019, 21, 60-64.
Courses taught by Hien Nguyen
Winter Term 2025 (future)
Fall Term 2024 (current)
Winter Term 2024
Fall Term 2023
Winter Term 2023
- CHM4850 - Frontiers in Chemistry
- CHM6220 - Organic Reactions and Synthesis
- CHM7220 - Organic Reactions and Synthesis
- CHM8850 - Frontiers in Chemistry
Fall Term 2022
- CHM8850 - Frontiers in Chemistry
- CHM4850 - Frontiers in Chemistry
- CHM5510 - Chemical Synthesis Laboratory